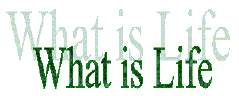
Neurotransmitters and hormones
Tissue specialization
of amino acid metabolism
KEGG
compound catalogue
The metabolism of amino acids serves as stage for the formation
of many small signaling molecules used as hormones and neurotransmitters.
Neurotransmitters are synthesized in neurons and act locally. They
are released into a synaptic cleft providing a diffusible chemical
signal from one cell to the next, cells which are not in direct
contact with each other. These synapses are therefore referred to
as chemical synapses, as opposed to electrical synapses, which use
a gap junction mediated coupling of action potentials without a
mediating chemical signaling mechanism. The signal transmission
of the latter is much faster than that found in chemical synapses.
Neurotransmitters bind to receptor proteins and channels and initiate
signaling events mainly related to electrical transmissions such
as action potentials, or stimulating calcium waves in the cytoplasm.
Some signaling molecules are not only used locally and thus serve
as hormones having a larger range of activity, like epinephrine,
which affects carbohydrate and lipid metabolism in various target
organs, far away from the site of synthesis. Other types include
thyroxin, an amino acid derivative produced in the thyroid gland
and important for organ differentiation, growth, and metamorphosis,
and nitric oxide, NO, a short lived free radical
gas that acts as hormone and neurotransmitter. Nitric oxide's role
in mammalian physiology had been discovered in the 1980s.
Tissue specialization is an important aspect of amino acid metabolism.
While neurotransmitters and hormones are synthesized in neurons
and specialized glands, it is the liver and skeletal muscle that
can utilize amino acid degradation for energy production, while
excess aromatic acids like phenylalanine are disposed by the kidney.
Tissue specialization for tryptophan produces glucose, ketone bodies,
and nicotinamide (for NAD synthesis) in the liver, serotonin in
neurons (mood control) and mast cells (allergy, inflammation), while
gastro intestinal (GI) bacteria break down tryptophan to provide
the host organism with indole ring precursors.
Albinism
The dark pigment melanin is synthesized from tyrosine in
the brain in melanocytes and secreted to the outer layer skin
epithelial cells. The lack of the enzyme tyrosinase
(EC 1.14.18.1; monophenol monooxygenase) causes albinism.
Partial albinism (leucism) also causes lack of pigmentation
(white alligator). Partial albinism is not a defect of tyrosinase,
but defects of melanocyte differentiation and migration.
Description of human albinism: Homozygotes lack tyrosinase
(TYR; EC 1.14.18.1) which catalyzes the first 2 steps, and
at least 1 subsequent step, in the conversion of tyrosine
to melanin
(C05606). This enzyme catalyzes at least four different reaction
steps. Incubation of hair bulbs with L-tyrosine or L-DOPA,
two intermediates in melanin formation, yields no melanin
supporting the evidence that the conversion needs tyrosine
as initial precursor. For a complete description of human
albinism at GenomeNet follow this link: albinism.
Information is also available at the National Center for Biotechnology
Information's OMIM
database (Online Mendelian Inheritance in Man
|
Fig. White (leucistic) alligator
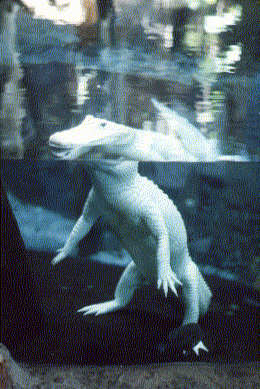
Photo © Lukas Buehler, 1991
Aquarium of the Americas, New Orleans |
Neurotransmitters
Neurotransmitters fall into two broad classes. The first group
consists of the amino acids aspartate, glutamate, its decarboxylated
form g -amino-butyric acid (GABA), and
glycine. The second group contains the biogenic amines acetylcholine,
serotonin, histamine, and the catecholamines epinephrine, nor-epinephrine,
and dopamine. Except for acetylcholine, all biogenic amines are
derived from aromatic amino acids tryptophan and tyrosine. To properly
understand the metabolism of neurotransmitters, one has to study
their 'life cycle' - (i) biosynthesis, (ii) storage, (iii) release,
and (iv) degradation or re-uptake.
Acetylcholine
Acetylcholine is a neurotransmitter found in cholinergic synapses
that provide a stimulatory transmission in the nervous system. Acetylcholine
(C01996) is synthesized from choline and acetyl-CoA by the
enzyme choline
acetyl transferase (EC 2.3.1.6) to form acetylcholine, which
is immediately stored in small vesicular compartments closely attached
to the cytoplasmic side of presynaptic membranes. Acetylcholine
synthesis is a process that occurs only in the specialized region
of neurons called synapses. The choline used for the synthesis of
acetylcholine is derived from the phospholipid phosphatidylcholine.
Enzymes called phospholipases catalyze the degradation of
phosphatidylcholine (PC).
Two independent pathways have been established for the release
of choline. First, phospholipase
D (EC 3.1.4.4) cleaves the phosphoester bond towards the
choline headgroup forming free choline and the membrane bound phosphatidic
acid (PA). Phospholipase D is predominantly localized to late endosomes
and lysosomes (FEBS Lett 1999; 442(2-3):221-5). Choline is then
covalently linked with an activated acetyl unit from acetyl-CoA
to form acetylcholine. This reaction is performed by choline-acetyl
transferase (EC 2.3.1.6). Second, PC is degraded into its
glycerol backbone and fatty acid components by the sequential action
of phospholipases A and B. Phospholipase
A1 (EC 3.1.1.32) or Phospholipase
A2 (EC 3.1.1.4) removes the acyl chain from the C1 position
forming a free fatty acid and lysophosphatidylcholine (lysolecithin).
The second phospholipase
B (EC 3.1.1.5) removes the C2 acyl residue to form glycerol-3-phospocholine
and a fatty acid. Glycerol-3-phospocholine is hydrolyzed to glycerol-3-phosphate
(G3P) and choline.
Acetylcholine is then accumulated and stored in synaptic vesicles
via a vesicular acetylcholine transporter. These vesicles
are closely associated with the cytoplasmic surface of the presynaptic
membrane. Phospholipase D may play a role in guiding these acetylcholine
loaded vesicle to the membrane. This is based on evidence that phospholipase
D activity is associated with control of membrane vesicular transport.
This lipase may thus provide a double mechanism for choline synthesis
and the cytoplasmic transport of acetylcholine containing vesicles.
Upon a stimulus from an action potential and mediated by calcium
induced membrane fusion (exocytosis), up to 300 vesicles per synapse
release their contents (acetylcholine) into the synaptic cleft,
instantly providing a high concentration of neurotransmitters. Acetylcholine
concentration temporarily raises from 10nM to 0.5mM, a 50,000 fold
increase occurring in about a millisecond. Acetylcholine rapidly
diffuses across the cleft (20 to 50 nm) binding to nicotinic
acetylcholine receptors located in the post-synaptic membranes
found at neuro-muscular junctions. These receptors initiate an action
potential event in the muscle cell membrane causing a massive influx
of extra-cellular calcium, thereby triggering muscle contractions.
The exocytotic process of acetylcholine release can be inhibited
by botulinum toxin. This potent toxin prevents the membrane
fusion process and causes muscle paralysis. Botulinum toxin is actually
a mixture containing eight distinct proteins produced by the anaerobic
bacteria Clostridium botulinum. So called botulism is a major
cause of food poisoning related to unrefrigerated meat.
Acetylcholine esterase
(AChE)
Acetylcholine is rapidly removed by acetylcholine
esterase (EC 3.1.1.7; AChE). The turnover rate of hydrolysis
is 2.5x104 molecules per second. This hydrolytic degradation
ensures that the signal does not overstimulate the post-synaptic
membrane. A molecular dynamics simulation published in 1994 in the
Journal 'Science' suggested that electrostatic fields funnel acetylcholine
into the active site channel and release the hydrolysis products
through a 'back door' (Science,
1994, 263(6161)1276). The products choline and acetate are inactive
molecules and are reabsorbed by the synapse and recycled to replenish
acetylcholine containing vesicles for subsequent chemical transmission.
AChE is a serine esterase and has a catalytic triad similar
to that found in serine proteases trypsin and chymotrypsin. The
triad consists of Ser200, His440, and Glu237 (note that serine proteases
contain a aspartate and not glutamate). The enzyme is located on
the surface of the post-synaptic membrane and linked by a GPI
anchor.
AChE can be inhibited resulting in the overstimulation of neuromuscular
junctions. This leads to spasms and death by suffocation because
the heart muscles experience severe arrhythmia. AChE inhibitors
have been used for a long time by the military as nerve gas. The
most prominent are tabun and sarin, which are specific
for the human acetylcholine esterase. An insect specific AChE inhibitor,
malathion, which does not bind to the human isoform of the enzyme,
is used by citrus crop producers to fight against Mediterranean
fruit fly (Medfly; Ceratitis capitata) infestations.
Glutamate and GABA
Glutamate
(C00025) and its decarboxylated derivative gamma aminobutyric
acid (GABA;
C00334) are the major excitatory and inhibitory neurotransmitters
in the central nervous system, respectively. Glutamate
decarboxylase (EC 4.1.1.15) is a pyridoxal-phosphate dependent
protein removing the alpha-carboxyl group from glutamate. GABA,
if not used as neurotransmitter, can be deaminated to form succinate
by an aminotransferase reaction involving the conversion of a-ketoglutarate
to glutamate. Thus, succinate can be generated from alpha-ketoglutarate
in a citric acid cycle by-pass reaction located in the cytoplasm
of neurons. This process is known as GABA shunt. (KEGG pathway
MAP00251
glutamate metabolism)
GABA is one of the brain's major inhibitory neurotransmitter (in
the CNS, the other is glycine, which is predominantly found in the
spinal cord) by activating a chloride selective receptor ion channel
(GABA receptor). The active phase of these chloride conducting channels
stabilizes the negative resting potential of the post-synaptic membrane,
counteracting any stimulatory activity by glutamate or acetylcholine.
Serotonin
The neurotransmitter serotonin
(C00780) is found in the brain, lung, and gastro intestinal (GI)
system. It is a major control substance of smooth muscle contractions.
In the central nervous system, serotonin is involved in fear and
flight responses, an activity which is opposed by the aggression
stimulating hormone adrenaline (epinephrine) and neurotransmitter
dopamine. Serotonin is derived
from tryptophan in two reaction steps. First, tryptophan is
hydroxylated by tryptophan
5-monooxygenase (EC 1.14.16.4) to form 5-hydroxy-tryptophan
(C00643). The net reaction of monooxygenase includes the coenzyme
tetrahydrobiopterine:
L-Tryptophan + Tetrahydrobiopterin + O2 =
5-Hydroxy-L-tryptophan + Dihydrobiopterin + H2O
5-hydroxytryptophan is decarboxylated by tryptophan
decarboxylase (EC 4.1.1.28) in a reaction analogous
to glutamate decarboxylase. Many decarboxylases are more
or less substrate specific. Tryptophan decarboxylase, a pyridoxal-phosphate
protein, acts on three different substrates including L-tryptophan,
5-hydroxy-L-tryptophan and dihydroxy-L-phenylalanine.
Serotonin, after being secreted into the synaptic cleft, is removed
from this extra-cellular location by an active (energy consuming)
reuptake mechanism, which pumps its back into the synapse. Thus,
serotonin is not degraded outside the cell, but by the mitochondrial
enzyme monoamine
oxidase (MAO; EC 1.4.3.4). The end
product of this degradative pathway is 5-hydroxyindolacetate
(C05634), which is not metabolized any further, but instead secreted
in the urine.
Lack of serotonin is often associated with depression. Restoring
the normal or enhanced level of this neurotransmitter acts as mood
enhancer. Prozac is a mood enhancing drug, which acts in the central
nervous system by inhibiting the reuptake mechanism of serotonin
into the synapse. Since serotonin is not degraded in the synaptic
cleft, Prozac promotes a prolonged presence of serotonin keeping
the post-synaptic membrane active. Inhibition of MAO also leads
to a prolonged activation of serotonergic synapses. Although the
molecular mechanism of reuptake inhibition is fairly well known,
the relationship between serotonin, or better its receptors (eight
different subtypes known thus far), and mental stability is not
understood.
Melatonin
Melatonin
(C01598), synthesized by the pineal gland and retina, is the chemical
messenger which allows seasonal animals including man to perceive
day length changes. It is derived from serotonin by an acetylation
catalyzed by serotonin
N-acetyltransferase (EC 2.3.1.87) to form acetylserotonin
(C00978). The latter is methylated to melatonin. The methyl group
is donated from S-adenosylmethionine and the reaction catalyzed
by N-methyltransferase
(EC 2.1.1.4).
Melatonin production is regulated by light through the retino-hypothalamic
tract. The N-acetyltransferase levels are under hormonal control.
It is the stimulatory effect of norepinephrine that activates gene
expression. Besides controlling sleep patterns, melatonin is also
involved in the modulation of mood, sexual behavior, reproductive
alterations, and immunological functions. It is also studied as
an anti-oxidant molecule in the blood. The critical (rate limiting)
step in its synthesis depends on N-acetyltransferase. It
has been found that the circadian rhythm is controlled by melatonin
blood plasma concentrations. The concentration at night is about
3x higher than during the day.
Melatonin uses its own receptors, Mel1 and Mel2. The short description
reproduced below the current findings on the structure and
function of melatonin receptors (by Jocker et al., C R Seances Soc
Biol Fil 1998;192(4):659-67):
Melatonin receptors belong to the super-family of G protein-coupled
receptors. They modulate a large spectrum of physiological functions
including regulation of circadian rhythms and seasonal reproduction.
Pharmacological evidence suggests the expression of two types
of receptors, called Mel1 and Mel2. So far, only Mel1 receptors
have been cloned and classified into three subtypes (Mel1A, Mel1B,
Mel1C). Mel1 receptors are expressed in the brain, the retina
and several other peripheral tissues.
Catecholamines
The neurotransmitter dopamine
(C03758) is a stimulatory neurotransmitter and often functions as
a physiological antagonist of serotonin, using its own receptors.
Dopamine is also a precursor for the synthesis of the hormones nor-epinephrine
(nor-adrenaline) and epinephrine (adrenaline). See KEGG pathway
MAP00350
for details.
Tyrosine is hydroxylated by monophenol
monooxygenase (EC 1.14.18.1) in a reaction similar to tryptophan
hydroxylase forming L-DOPA
(C00355). This enzyme also catalyzes the formation of melanin (see
albinism). Here we have a neuron specific
isoform of the monooxygenase. The decarboxylation of L-DOPA is catalyzed
by EC 4.1.1.25 or tyrosine
decarboxylase. Dopamine is hydroxylated by dopamine
beta-monooxygenase (EC 1.14.17.1) to nor-adrenaline
(C00547), which is methylated in the presence of S-Adenosyl-L-methionine
by noradrenaline
N-methyltransferase (EC 2.1.1.28) to form adrenaline
(C00788).
Note that while dopamine is a neurotransmitter of the central and
peripheral nervous system and thus acts as a local chemical messenger,
both adrenaline and nor-adrenaline are hormones and are produced
in the adrenal medulla containing the corresponding tissue specific
enzymes. This is the reason why tyrosine monooxygenase
(EC 1.14.18.1) can form either the catecholamines in neurons or
adrenal medulla, or melanin in skin epithelia. It is important to
keep in mind that the complicated pathways presented in KEGG like
the one for tryptophan metabolism does not exist in all cells due
to cell type specific activation or inhibition of gene expression.
Thus an intermediate like L-DOPA does not accumulate in the adrenal
medulla.
Thyroxine
The thyroid gland is a specialized organ that releases the growth
hormone thyroxine. Thyroxine
(C01829) is synthesized
from the aromatic amino acid tyrosine by multiple iodination,
rearrangement and hydrolysis steps. The process occurs on the specialized
protein thyroglobin using the protein's tyrosine residues as substrate.
Thyroglobin contains about 140 tyrosine residues.
Tyrosine residues are iodinated at position 3 and 5 of the
benzene ring to form the intermediates 3-iodo-L-tyrosine and 3,5-diiodo-L-tyrosine.
The enzyme catalyzing the reaction is called iodoperoxidase
(EC 1.11.1.8). Two diiodo-tyrosine residues are linked through an
ether bond forming thyroxine, which at this point is still part
of thyroglobin. During the rearrangement, the mechanism of which
is not fully elucidated, epoxide intermediates are likely to form.
Note that all reaction steps are catalyzed by the thyroid specific
peroxidase.
Tyrosine residues next to each other on the surface of thyroglobin
are first iodinated and then covalently linked through a ether bridge.
The rearrangement reaction involves the formation of possibly epoxide
intermediates. The exact reaction mechanism is not fully understood.
Research on non-protein bound tyrosine yields reactive epoxide
intermediates with structures that may resemble the intermediates
found during thyroxine synthesis on thyroglobin.
Thyroglobin linked thyroxine units are not physiologically active.
Thyroglobin acts as a thyroxine storage device and the proteolysis
acts as control mechanism of hormone activation. Thyroglobin is
proteolytically degraded (peptide bond hydrolysis) and free thyroxine
units are released. This free thyroxine is subsequently secreted
into the blood plasma where it is bound to plasma proteins like
globins, pre-albumin and albumin.
Thyroxine enters target cells where it controls gene expression
by binding to nuclear receptor proteins (transcription factors in
eukaryotic cell nuclei). It acts as a morphogen by controlling the
growth and differentiation of cells. For example, it has been identified
as the hormone controlling metamorphosis of tadpoles to frogs.
Go to table of contents
|