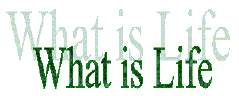
Nucleic acid metabolism
Pyrimidine and purine
metabolism
(KEGG pathway overview MAP01140)
Nucleic acids are important intracellular signaling molecules and
coenzymes, are the single most important means of coupling endergonic
to exergonic reactions, and are the storage of genetic information
in the form of DNA and RNA. The latter is composed of nucleotides
containing a phosphoribosyl component and either one of the aromatic
base adenine (A), guanine (G), cytosine (C), and uracyl (U). These
bases can be distinguished by their nitrogen containing, aromatic
ring structures and come in two forms - pyrimidines
and purines
.
Nucleotides are derived from biosynthetic precursors of carbohydrate
and amino acid metabolism, and from ammonia and carbon dioxide.
The liver is the major organ of de novo synthesis of all four
nucleotides. Degradation in humans, however, is only complete for
pyrimidines (C, T, U), but not purines (G, A), which are excreted
from the body in form of uric
acid (C00366). De novo synthesis of pyrimidines and purines
follows two different pathways. Pyrimidines are synthesized first
from aspartate and carbamoyl-phosphate in the cytoplasm to the common
precursor ring structure orotic
acid (C00295), onto which a phosphorylated ribosyl unit
is covalently linked. Purines, however, are first synthesized from
the sugar template onto which the ring synthesis occurs.
Pyrimidine synthesis
(KEGG pathway MAP00240)
The synthesis of the pyrimidines CTP and UTP occurs in the cytoplasm
and starts from the condensation of aspartate with carbamoyl-phosphate
to form orotic acid. The enzyme Aspartate
carbamoyltransferase (EC 2.1.3.2) forms N-carbamoyl-aspartate
(C00438) which is converted into dihydroorotic
acid (C00337) by Dihydroorotase
(EC 3.5.2.3). The latter is converted to orotate
(C00295) by Dihydroorotate
oxidase (EC 1.3.3.1). The net reaction is:
(S)-Dihydroorotate + O2 = Orotate + H2O2
The formation of cytidine triphosphate in E.coli is controlled
by feedback (end product) inhibition. As a critical concentration
of CTP is building up, CTP slows down its own formation by inhibiting
Aspartate carbamoyltransferase. This enzyme is composed of
two large subunits, one of which carries the catalytic site and
the other the regulatory site.
Orotic acid is covalently linked with a phosphorylated ribosyl
unit. The activated precursor is PRPP
(C00119; 5-Phosphoribosyl 1-pyrophosphate). The covalent linkage
between the ribose and pyrimidine occurs at position C1 of the ribose
unit, which contains a pyrophosphate, and N1 of the pyrimidine
ring. Orotate
phosphoribosyltransferase (EC 2.4.2.10) catalyzes the net
reaction:
Orotate + 5-Phospho-alpha-D-ribose 1-diphosphate =
Orotidine 5'-phosphate + Pyrophosphate
Orotidine-5-phosphate
(C01103) is decarboxylated by Orotidine-5'-phosphate
decarboxylase (EC 4.1.1.23). The enzyme from higher eucaryotes
is identical with EC
2.4.2.10, meaning that the PRPP transferase catalyzes both the
ribosylation and decarboxylation reactions, forming UMP from orotic
acid in the presence of PRPP. The monophosphonucleotide is phosphorylated
by two kinases. First the diphosphate form UDP is produced, which
in turn is phosphorylated to UTP. Both steps are fueled by ATP hydrolysis:
ATP + UMP = ADP + UDP
UDP + ATP = UTP + ADP
CTP is subsequently formed by amination of UTP by the catalytic
activity of CTP
synthetase (EC 6.3.4.2). Glutamine is the NH3 donor and
the reaction is fueled by ATP hydrolysis, too:
UTP + Glutamine + ATP + H2O = CTP + ADP + Pi
Purine synthesis
(KEGG pathway
MAP00230)
Purine ring synthesis starts from the activated ribose PRPP with
the sequential addition of nitrogen and carbon containing units,
donated from either glutamine (N), glycine (N&C), aspartate
(N), folic acid (C1), or CO2.
1. |
This
is the committed step in purine synthesis: glutamine donates
an NH2 group displacing the pyrophosphate at ribose C1. |
2. |
Next,
a glycine is incorporated fueled by ATP hydrolysis. The carboxyl
group forms an amine bond to the NH2 introduced in step 1. |
3. |
A one-carbon unit from folic acid coenzyme N10-formyl-THF is
added to the amino group of the substituted glycine followed
by the closure of the imidazole ring. |
4. |
Next,
a second NH2 group is transferred from a glutamine to the first
carbon of the glycine unit. |
5. |
A
carboxylation of the second carbon of the glycin unit is concomittantly
added. |
6. |
This
new carbon is modified by the additional of a third NH2 unit,
this time transferred from an aspartate residue. |
7. |
Finally,
a second one-carbon unit from formyl-THF is added to the nitrogen
group and the ring covalently closed to form the common purine
precursor inosine monophosphate (IMP;
C00130). |
Because of the addition of one-carbon units, nucleic acid synthesis
is strictly dependent on folic acid (vitamin). Folic acid is the
precursor for the activated one-carbon unit donor tetrahydrofolate
(THF). The major source of carbon units for nucleic acid synthesis
comes from serine. In this reaction, serine is converted to glycine,
while one methylene group is covalently linked to THF to form 5,10-methylene-tetrahydrofolate.
This reaction is catalyzed by a mitochondrial enzyme. For more details
see one
carbon pool metabolism.
Inosine monophosphate is converted to adenosine monophosphate in
two steps. First, GTP hydrolysis fuels the addition of aspartate
to IMP by adenylosuccinate
synthase (EC 6.3.4.4), substituting the carbonyl oxygen
for a nitrogen and forming the intermediate adenylosuccinate. Fumarate
is cleaved off forming adenosine monophosphate. This step is catalyzed
by adenylosuccinate
lyase (EC 4.3.2.2).
GMP is formed by oxidation of IMP forming xanthylate, followed
by the insertion of an amino group at C2. NAD+ is the electron
acceptor in the oxidation reaction. The amide group transfer from
glutamine is fueled by ATP hydrolysis.
Purine synthesis is regulated by feed back inhibition by the end
products. Analogous to amino acid synthesis, the IMP branching point
is regulated. The branch leading to AMP is inhibited by AMP, the
branch leading to GMP is inhibited by GMP. Note that the catalytic
steps are fueled by nucleotide hydrolysis in a reciprocal fashion.
GTP is used for AMP formation and ATP is used for GMP formation.
This way, the cell has a control over the relative levels synthesize
for each nucleotide.
Uric acid formation
While pyrimidine rings can be degraded completely to CO2 and NH3
(urea excretion), purine rings are degraded to the metabolically
inert uric
acid (C00366). GMP is split into the base guanine and ribose.
Guanine is deaminated to xanthine which in turn is oxidized to uric
acid. This last reaction is irreversible. Similarly, AMP is deaminated
to IMP from which the ribose unit is removed to form hypoxanthine.
Hypoxanthine is oxidized to xanthine and finally to uric acid. Instead
of uric acid secretion, guanine and IMP can be used for recycling
purposes and nucleic acid synthesis in the presence of PRPP and
aspartate (NH3 donor).
If the proper disposal of uric acid is impaired, it can cause inflammations,
joint pain (arthritis), and kidney problems. This is a condition
called gout and is enhanced by low pH conditions, where uric acid
is less soluble and starts to crystallize. Gout can be cause by
enzymatic defects in guanine recycling and the accumulation of xanthine,
which leads to excess uric acid synthesis.
Formation of deoxyribonucleotides
for DNA synthesis
Deoxyribonucleotides are synthesized from their ribonucleotide-diphosphates
(DNP) by reduction of their C2 ribose carbon. Enzymes catalyzing
this reaction are called ribonucleotide
reductases (diphosphate reductase EC 1.17.4.1). The 2'-OH
hydroxyl-group of ribose is replaced by a hydrogen atom (H) via
a free radical reaction.
The reaction steps of the E.coli
class I enzyme can be summarized as follows:
1.
|
The
active site of the enzyme contains two tyrosine radicals that
are stabilized by binuclear Fe-O2-Fe complexes. The tyrosine
free radicals abstract an H atom from the C3' position of the
diphosphate nucleotide substrate (DNP), forming a free radical
substrate intermediate. |
2.
|
Acid
catalyzed cleavage of the C2'-OH bond involving a sulfhydryl
acid-base reaction releases an H2O molecule and a radical-cation
intermediate (DNP+). |
3.
|
The
radical-cation intermediate is reduced by a second cystein pair
to yield 3'-deoxynucleotide radical intermediate. |
4.
|
This
3' radical abstracts the hydrogen from the tyrosine restoring
the initial free radical tyrosine active site and the reduced
2'-deoxyribonucleotide (dDNP). |
5.
|
The
reductase is now in an oxidized state with a cysteine pair,
which has to be reduced to regenerate the initial free cysteine
sulfhydryls. This regeneration of the reduced form of ribonucleotide
reductase is controlled by thioredoxin, a monomeric,
small protein (108 amino acids), which contains a pair of closely
spaced cysteine residues. Reduced thioredoxin reduces oxidized
reductase (it donates reducing equivalents to the cystein pairs
on the reductase). Thioredoxin is regenerated by thioredoxin
reductase (EC 1.6.4.5), which transfers electrons from
its FADH2 coenzyme to thioredoxin. The primary electron donor
in this reaction is NADPH. |
Regulation of ribonucleotide reduction is controlled by allosteric
feedback mechanisms. The levels of dATP inhibits binding of all
NDPs to the reductase. In addition, there is a multiple cross
over inhibition and activation of all dNTP molecules promoting the
even synthesis of DNA precursors. The absence of any of these dNTPs
is lethal for cells.
Synthesis of dTMP
DNA contains a nucleotide base component not found in RNA. This
is thymine and is derived from methylation of dUMP to dTMP
(C00364). The deoxyuracylmonophosphate is first formed by dephosphorylation
from dUTP by dUTP
diphosphorylase (EC 3.6.1.23). dTMP is then synthesized
from dUMP by thymidylated
synthase (TS; EC 2.1.1.45) with N5,N10-methylene-THF as
the methyl donor.
During this methylene transfer, the CH2 is reduced to a methyl
group at the expense of the oxidation of tetrahydrofolate (THF)
to dihydrofolate (DHF). This mechanism is distinctly different from
the 'simpler' methyl group transfer reaction using N5-methyl-THF.
DHF needs to be reduced in order to be recycled with an activated
one carbon unit. The levels of the amino acid serine and glycine
are linked to nucleic acid synthesis showing again the close inter-relationship
between the metabolism of the four major macromolecular systems
in living organisms.
Go to table of contents
|